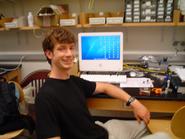
Lasers are versatile tools that have many applications in research and industry. Improving laser technology, therefore, is a top priority for those who use or produce equipment that utilizes lasers. At Hamilton, Professor of Physics Ann Silversmith has focused her research on developing new laser materials that would be useful in the solid-state laser industry. This summer Dan Campbell '08 (Pittsford, N.Y.) has been working with her to study solid-state lasers that use sol-gel encapsulated rare earth metals as their laser material.
According to Campbell, the encapsulated rare earth metals he and Silversmith are developing would offer numerous advantages over traditional laser materials because "they don't break down as easily, they are less finicky, and they are safer." In addition, these lasers are very compact, allowing "you to fit many different lasers into one small package and thus cover more of the light spectrum." The term laser, an acronym for "light amplification by stimulated emission of radiation," describes devices that emit a narrow, intense beam of light. Lasers operate on the principle of atom excitation. If energy (i.e. heat, light, electricity, etc.) is applied to an atom, it will leave its usual low-energy ground state and go to a high-energy excited state (this excitation corresponds to electrons moving from a lower energy level to a higher energy level). As the excited atom returns to the ground state (i.e. its electrons move back to a lower energy level), it releases the excess energy it absorbed in the form of a photon (i.e. particle of light).
Lasers control the way that excited atoms release their photons. Atoms in the laser material are "pumped" with energy (typically with light or electrical discharges) so the majority of them will enter the excited state. As atoms begin to return to the ground state, the photons they emit can stimulate other excited atoms to release light of the same frequency and phase. Mirrors within the laser cause this emitted light to bounce back and forth within the laser material so that more and more atoms relax and produce light. This cascade effect propagates many, many photons of the same frequency and phase, which eventually leave the laser as a beam of monochromatic, coherent, and intense light.
For his laser material, Campbell is working with the rare earth metals of the Lanthanide series (the 15 elements with atomic numbers 57-71 at the bottom of the periodic table), which are characterized by an unfilled 4f electron shell (an electron shell indicates the probability of finding the electrons that surround the nucleus of an atom in a certain area of space). To excite the rare earth metal atoms, Campbell shines a UV light with a specific energy onto the laser material (since only certain excitations result in photons, the energy of the light corresponds to the energy needed for the atoms to enter these desired excited states). As the electrons return to the ground state, "the electrons give up heat or vibrational energy until they reach some 4f to 4f transition that is too big to jump with vibrations or heat." It is at this point that a photon is emitted and the excited atoms fluoresce, returning them to the ground state. What is unique about the Lanthanide elements, however, is that in their first few excited states the electrons do not leave their 4f energy shell for a higher energy level. Instead, they remain in the 4f shell and simply make 4f to 4f transitions. Since the unfilled 4f subshells are closer to the nucleus than other filled higher energy subshells, a rare earth making these transitions in a glass will fluoresce in the same way as if it were in a crystal.
The rare earth metals used in the laser material are contained within sol-gel derived silica glass, an optically transparent, porous material. When the sol-gel is still a liquid, it is mixed with the rare earth metals. As the glass begins to harden into a solid, the Lanthanide elements are trapped inside. By putting the rare earth metals in sol-gel glass, Campbell is able to increase the intensity of their fluorescence. In normal glass, the atoms tend to cluster together. Consequently, when they are excited, the atoms prefer to share the energy with each other rather than emit light. The atoms encapsulated in sol-gel, however, are less prone to this clustering. This property of the silica glass allows Campbell to use much higher concentrations of rare earth metals than would be possible in normal glass, which translates to much brighter, more intense fluorescence when the atoms are excited.
One problem Campbell has encountered during his research is the presence of water in the laser material. This water dims the fluorescence because it is able to accept the excess energy from the excited rare earth metals, preventing them from emitting photons. Campbell is currently studying how water gets into the sol-gel. Does it get trapped inside the glass with the rare earth metals or does it solely enter through the sol-gel's pores? Once he understands how water gets in, he will hopefully be able to devise a method of removing it. Campbell is also studying how the rare earth metals are trapped inside the sol-gel. Are they actually encapsulated within the glass or are they deposited in the pores while still remaining in solution?
This is Campbell's third year of summer research, and he plans on making his current project into his senior thesis. A physics major and possible math minor, he wants to someday become a physics professor after attending graduate school. Campbell is a member of the orchestra and is the president of the Hamilton Capoeira Club.
-- by Nick Berry '09
According to Campbell, the encapsulated rare earth metals he and Silversmith are developing would offer numerous advantages over traditional laser materials because "they don't break down as easily, they are less finicky, and they are safer." In addition, these lasers are very compact, allowing "you to fit many different lasers into one small package and thus cover more of the light spectrum." The term laser, an acronym for "light amplification by stimulated emission of radiation," describes devices that emit a narrow, intense beam of light. Lasers operate on the principle of atom excitation. If energy (i.e. heat, light, electricity, etc.) is applied to an atom, it will leave its usual low-energy ground state and go to a high-energy excited state (this excitation corresponds to electrons moving from a lower energy level to a higher energy level). As the excited atom returns to the ground state (i.e. its electrons move back to a lower energy level), it releases the excess energy it absorbed in the form of a photon (i.e. particle of light).
Lasers control the way that excited atoms release their photons. Atoms in the laser material are "pumped" with energy (typically with light or electrical discharges) so the majority of them will enter the excited state. As atoms begin to return to the ground state, the photons they emit can stimulate other excited atoms to release light of the same frequency and phase. Mirrors within the laser cause this emitted light to bounce back and forth within the laser material so that more and more atoms relax and produce light. This cascade effect propagates many, many photons of the same frequency and phase, which eventually leave the laser as a beam of monochromatic, coherent, and intense light.
For his laser material, Campbell is working with the rare earth metals of the Lanthanide series (the 15 elements with atomic numbers 57-71 at the bottom of the periodic table), which are characterized by an unfilled 4f electron shell (an electron shell indicates the probability of finding the electrons that surround the nucleus of an atom in a certain area of space). To excite the rare earth metal atoms, Campbell shines a UV light with a specific energy onto the laser material (since only certain excitations result in photons, the energy of the light corresponds to the energy needed for the atoms to enter these desired excited states). As the electrons return to the ground state, "the electrons give up heat or vibrational energy until they reach some 4f to 4f transition that is too big to jump with vibrations or heat." It is at this point that a photon is emitted and the excited atoms fluoresce, returning them to the ground state. What is unique about the Lanthanide elements, however, is that in their first few excited states the electrons do not leave their 4f energy shell for a higher energy level. Instead, they remain in the 4f shell and simply make 4f to 4f transitions. Since the unfilled 4f subshells are closer to the nucleus than other filled higher energy subshells, a rare earth making these transitions in a glass will fluoresce in the same way as if it were in a crystal.
The rare earth metals used in the laser material are contained within sol-gel derived silica glass, an optically transparent, porous material. When the sol-gel is still a liquid, it is mixed with the rare earth metals. As the glass begins to harden into a solid, the Lanthanide elements are trapped inside. By putting the rare earth metals in sol-gel glass, Campbell is able to increase the intensity of their fluorescence. In normal glass, the atoms tend to cluster together. Consequently, when they are excited, the atoms prefer to share the energy with each other rather than emit light. The atoms encapsulated in sol-gel, however, are less prone to this clustering. This property of the silica glass allows Campbell to use much higher concentrations of rare earth metals than would be possible in normal glass, which translates to much brighter, more intense fluorescence when the atoms are excited.
One problem Campbell has encountered during his research is the presence of water in the laser material. This water dims the fluorescence because it is able to accept the excess energy from the excited rare earth metals, preventing them from emitting photons. Campbell is currently studying how water gets into the sol-gel. Does it get trapped inside the glass with the rare earth metals or does it solely enter through the sol-gel's pores? Once he understands how water gets in, he will hopefully be able to devise a method of removing it. Campbell is also studying how the rare earth metals are trapped inside the sol-gel. Are they actually encapsulated within the glass or are they deposited in the pores while still remaining in solution?
This is Campbell's third year of summer research, and he plans on making his current project into his senior thesis. A physics major and possible math minor, he wants to someday become a physics professor after attending graduate school. Campbell is a member of the orchestra and is the president of the Hamilton Capoeira Club.
-- by Nick Berry '09