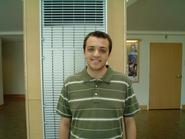
One of the major challenges facing modern physicists is trying to reconcile the seemingly incompatible theories of quantum mechanics, which describes three of the fundamental forces of nature (electromagnetic, weak nuclear, and strong nuclear forces), with general relativity, the theory of the fourth fundamental force (gravity).
Put more simply, quantum mechanics provides a model for understanding the structure and behavior of atoms and molecules while general relativity explains the phenomenon of gravity, which dominates the large scale. Much of the difficulty in merging these theories at all size scales comes from the different assumptions that these theories make on how the universe works. Nevertheless, scientists would like to create a so-called "theory of everything" to unite the physics of the atomic world with that of the macroscopic one and to provide a unified framework for understanding all four fundamental forces. Two of the theories proposed by scientists to accomplish this task are string theory and loop quantum gravity. This summer, Michael Gregg '08 (Albany, N.Y.), a physics major and creative writing minor, is working with Associate Professor of Physics Seth Major to study the latter theory.
Treating gravity as simply another quantum force has been difficult because, according to Einstein, gravity is not a force but a property of space-time itself. Loop quantum gravity (LQG) is an attempt to develop a quantum theory of gravity based directly on Einstein's formulations. In its most general sense, LQG is a field devoted to finding the microstructure of space-time and attempts to describe physics at the smallest possible scale, the Planck length (~10^(-35)m). If space is a continuum, as general relativity says it is, then in every part of it one can define regions of arbitrarily small volume. In LQG, the basic structure of space turns out to be discrete; for instance, area is quantized in units of Planck length squared. Just as with Legos you can't build a structure smaller than your smallest Lego block, space can't exist in any smaller quantity than these Planck scale units. However, remember how small this scale is: one fundamental unit is as small compared to a proton (one of the subatomic particles that make up atoms) as a proton is to us. LQG represents these discrete bits of space as a network of interconnected loops just as fields are used to represent electricity.
Last summer, Gregg used LQG to try to explain the anomalous acceleration of the Pioneer spacecraft. Specifically, he attempted to determine if traveling through the discrete spacetime predicted by LQG would result in the spacecraft's aberrant movement. Now, he is applying LQG to calculations involving white dwarf stars, which is what stars like our Sun become after they have exhausted their nuclear fuel. Subrahmanyan Chandrasekhar, an Indian physicist, developed a mathematical model for calculating the maximum mass that can be supported against gravitational collapse by electron degeneracy pressure, a quantum mechanical force that resists electrons being squeezed together. Electron degeneracy pressure will halt the gravitational collapse of a star if its mass is below 1.4 solar masses (1 solar mass is the mass of our Sun). A star exceeding this limit without usable nuclear fuel, however, will continue to collapse to form a neutron star or black hole because the degeneracy pressure provided by the electrons is weaker than the inward pull of gravity.
Since electron degeneracy pressure supports white dwarfs and prevents them from collapsing, 1.4 solar masses is the upper mass limit these stars can theoretically obtain and still remain stable. The force caused by the electron degeneracy pressure has a certain energy value associated with it, and Major, collaborating with Tomasz Konopka '02, had previously devised a modification to this energy to take into account the effects of loop quantum gravity. Gregg's ultimate goal this summer is to take these modified energy relations, assimilate them into the mathematical formulas for the upper mass limit of white dwarf stars, and determine whether the new pressure exerted by the electrons would be able to support a greater or smaller maximum mass than predicted by Chandrasekar's limit.
Thus far, Gregg has found his research to be a unique experience because it involves purely theoretical work. Rather than perform an actual experiment, he must look at a theory (LQG) that is not well understood and try to understand what effects its predictions would have on a variety of topics in physics. Gregg is still in the process of learning about Chandrasekhar's mathematical model explaining the upper mass limits of stars, and, as a result, his research is still in its early stages. He plans to continue the project during the school year as his senior thesis. On campus, Gregg is the co-chair of HamPoll, a student polling organization.
-- by Nick Berry '09
Put more simply, quantum mechanics provides a model for understanding the structure and behavior of atoms and molecules while general relativity explains the phenomenon of gravity, which dominates the large scale. Much of the difficulty in merging these theories at all size scales comes from the different assumptions that these theories make on how the universe works. Nevertheless, scientists would like to create a so-called "theory of everything" to unite the physics of the atomic world with that of the macroscopic one and to provide a unified framework for understanding all four fundamental forces. Two of the theories proposed by scientists to accomplish this task are string theory and loop quantum gravity. This summer, Michael Gregg '08 (Albany, N.Y.), a physics major and creative writing minor, is working with Associate Professor of Physics Seth Major to study the latter theory.
Treating gravity as simply another quantum force has been difficult because, according to Einstein, gravity is not a force but a property of space-time itself. Loop quantum gravity (LQG) is an attempt to develop a quantum theory of gravity based directly on Einstein's formulations. In its most general sense, LQG is a field devoted to finding the microstructure of space-time and attempts to describe physics at the smallest possible scale, the Planck length (~10^(-35)m). If space is a continuum, as general relativity says it is, then in every part of it one can define regions of arbitrarily small volume. In LQG, the basic structure of space turns out to be discrete; for instance, area is quantized in units of Planck length squared. Just as with Legos you can't build a structure smaller than your smallest Lego block, space can't exist in any smaller quantity than these Planck scale units. However, remember how small this scale is: one fundamental unit is as small compared to a proton (one of the subatomic particles that make up atoms) as a proton is to us. LQG represents these discrete bits of space as a network of interconnected loops just as fields are used to represent electricity.
Last summer, Gregg used LQG to try to explain the anomalous acceleration of the Pioneer spacecraft. Specifically, he attempted to determine if traveling through the discrete spacetime predicted by LQG would result in the spacecraft's aberrant movement. Now, he is applying LQG to calculations involving white dwarf stars, which is what stars like our Sun become after they have exhausted their nuclear fuel. Subrahmanyan Chandrasekhar, an Indian physicist, developed a mathematical model for calculating the maximum mass that can be supported against gravitational collapse by electron degeneracy pressure, a quantum mechanical force that resists electrons being squeezed together. Electron degeneracy pressure will halt the gravitational collapse of a star if its mass is below 1.4 solar masses (1 solar mass is the mass of our Sun). A star exceeding this limit without usable nuclear fuel, however, will continue to collapse to form a neutron star or black hole because the degeneracy pressure provided by the electrons is weaker than the inward pull of gravity.
Since electron degeneracy pressure supports white dwarfs and prevents them from collapsing, 1.4 solar masses is the upper mass limit these stars can theoretically obtain and still remain stable. The force caused by the electron degeneracy pressure has a certain energy value associated with it, and Major, collaborating with Tomasz Konopka '02, had previously devised a modification to this energy to take into account the effects of loop quantum gravity. Gregg's ultimate goal this summer is to take these modified energy relations, assimilate them into the mathematical formulas for the upper mass limit of white dwarf stars, and determine whether the new pressure exerted by the electrons would be able to support a greater or smaller maximum mass than predicted by Chandrasekar's limit.
Thus far, Gregg has found his research to be a unique experience because it involves purely theoretical work. Rather than perform an actual experiment, he must look at a theory (LQG) that is not well understood and try to understand what effects its predictions would have on a variety of topics in physics. Gregg is still in the process of learning about Chandrasekhar's mathematical model explaining the upper mass limits of stars, and, as a result, his research is still in its early stages. He plans to continue the project during the school year as his senior thesis. On campus, Gregg is the co-chair of HamPoll, a student polling organization.
-- by Nick Berry '09
Posted June 21, 2007